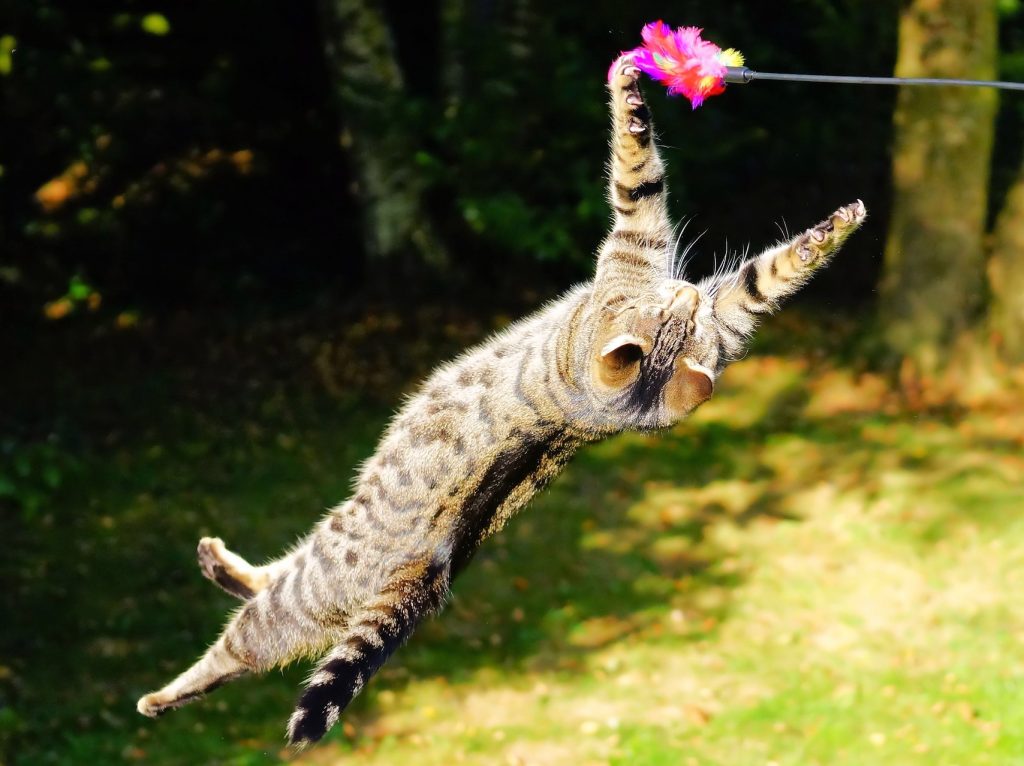
“Oh my goodness, she’s adorable!” Dr. Tanaka exclaimed, as Simon lifted the squirming kitten from its carrying case, nervously placing her onto the examination table.
“She is, isn’t she?” replied Simon. “I decided to name her Grace. Are you excited for your first visit to the vet, Grace?” Simon asked, directing his question toward the kitten.
“That’s a lovely name,” said Dr. Tanaka smiling. “The last time you were here, didn’t you say you had switched to a pre-veterinary major? How’s that going?” Just as the words left her mouth, the kitten had run to the edge of the table, diving headfirst into a roll that pitched her right off the table. By the time Simon shot out his hands in a futile attempt to catch her, she had landed feet first on the floor. “Well, she may not be graceful, but at least she’ll always land on her feet!” Dr. Tanaka joked, lifting her back onto the table and placing a toy in front of her.
“Ha ha. Well, my studying goes well when I’m not distracted by this one,” Simon replied, pointing at the kitten, now thoroughly distracted by the toy. “Actually, you must know the answer to this,” Simon said, turning back to Dr. Tanaka. “And I’m really curious now that I’ve just started taking anatomy and physiology- why do cats always land on their feet but humans don’t?”
“Well, cats don’t always land on their feet. But that expression does have a bit of truth to it. Cats are exceptionally flexible. As they’re falling they can twist their torso midair to get their feet below them before they reach the ground,” said Dr. Tanaka, her hands palm down in front of her to illustrate. “It’s called the ‘righting reflex.’”
“Oh wow. So humans don’t have that reflex then?” asked Simon.
“Well, humans aren’t as flexible as cats and we’re larger, giving us less time to reorient our body before we land. But actually we also have a righting reflex. In fact, we have the same sense of balance and ability to sense when we’re moving or what’s up versus down as a cat. It’s called the vestibular system. And it’s found here,” Dr. Tanaka continued, cupping her open hand around her ear to demonstrate, “in the ear or the back of the jaw in all vertebrates, or animals with a backbone.”
“Wait, are you saying that our ears give us our sense of balance? Maybe this is a silly question but I thought that ears were just for hearing?” asked Simon.
“That’s not a silly question and I’d be happy to explain it to a young veterinarian in training!” Dr. Tanaka replied, smiling as she turned to a small dry erase board on the wall of the veterinary clinic exam room. “Before we get to our sense of balance, we first have to talk about how our body senses that it’s moving.”
Anatomy of the ear
Taking a dry erase marker from the tray below the board, Dr. Tanaka started drawing. “Let’s start with the part of the ear you can see and work our way inwards. The ear is divided into 3 sections, the outer, middle, and inner ear. The outer ear is made up of the floppy part you can see, called the pinna, and the tunnel it connects to, called the external auditory canal. And the middle ear is where sound waves are processed. At the end of the auditory canal, sound waves hit and vibrate the tympanic membrane.”
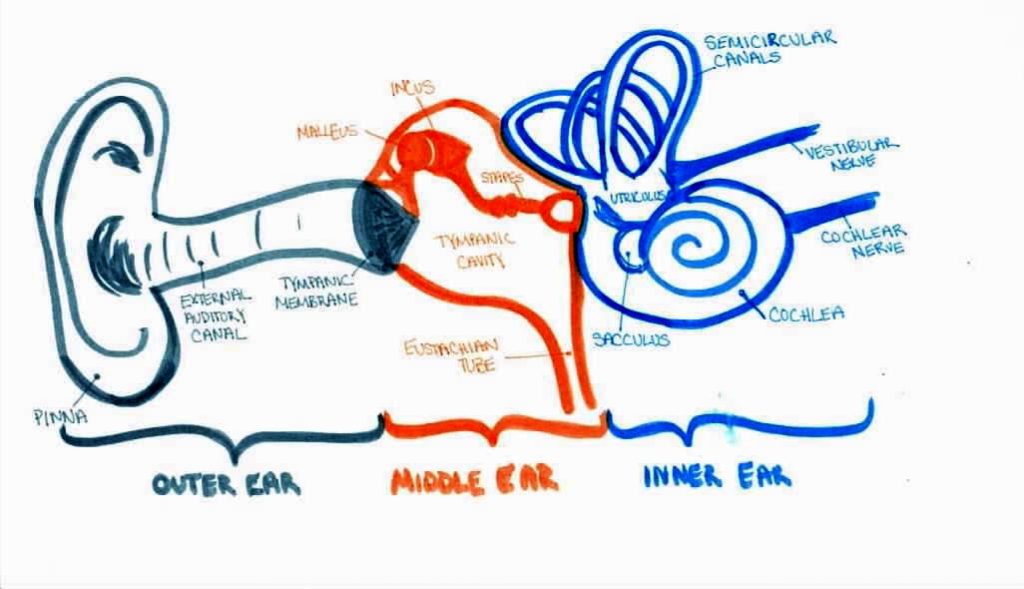
“Like a tympani in an orchestra?” Simon asked.
“Exactly, you’ve probably heard of this structure by its more common name, the eardrum,” Dr. Tanaka replied.
“Ah, right. What a great name!” Simon said.
“Sometimes anatomical terms can be quite creative,” Dr. Tanaka replied, smiling. “On the other side of the eardrum is the tympanic cavity, where the three ear bones are located. These are the malleus, incus, and stapes. Mammals are the only animals that have three bones in their ear. In other vertebrates, there’s just one bone in the middle ear, the stapes. These other two bones,” Dr. Tanaka continued, pointing to the malleus and incus, “are part of the jaw.”
“Wait, other vertebrates have ear bones in their jaw?” Simon asked, his eyes opened wide with surprise.
“It’s really the other way around,” Dr. Tanaka replied, “mammals have taken jaw bones and turned them into ear bones. The jaw and ear are adjoining structures and earlier in evolution our jaws were made up of several different bones. So when we needed additional bones in our ear, the jaw was a great structure to pilfer them from.”
“Evolution is crazy,” remarked Simon, shaking his head.
“It is, indeed,” replied Dr. Tanaka. “The last part of the middle ear is the eustachian tube, which connects to our throat and helps to keep the pressure in the middle ear the same as the pressure outside of the ear.”
“Wait, is that related to popping your ears? Like when you’re in a plane and you hold your nose and blow?” Simon asked.
“Yes, it is! The eustachian tube is collapsed most of the time since the walls are just soft tissue,” Dr. Tanaka said, bringing the palms of her hands together in front of her to demonstrate. “When you hold your nose to pop your ears you are sealing off your throat with your lips and tongue and then pushing air into your throat with your cheeks. This forces the eustachian tube open and makes the pressure in the middle ear the same as the outside pressure.”
“So, does that mean your throat is actually connected to your middle ear?” asked Simon.
“Yes! If you had a small enough wire you could actually insert a wire into your throat, pass it through the eustachian tube and touch the malleus, incus, and stapes of the middle ear. It’s all one space.”
“Wow, I can see now how the jaw and ear really are connected,” Simon said, moving his hand to the part of his cheek just in front of his ear as if to confirm it for himself.
“Those former jaw bones, now middle ear bones, transmit the vibrations from the eardrum to part of the inner ear, called the cochlea, that is filled with fluid and lined with tiny hair cells.”
“Wait, there’s hair inside my cochlea?” asked Simon.
“No, not like the hair on your head,” Dr. Tanaka replied smiling. “These are tiny microscopic projections that grow out of the surface of a cell called stereocilia, like the microvilli in your gut. But since they look like hair growing out of a cell, they’re called hair cells. As sound moves through the fluid in the cochlea it causes the fluid and membranes within the cochlea to move and this causes the hair of the hair cells to bend. Neurons connect to these hair cells and every time the hairs bend, the neurons send a signal to the brain, via the cochlear nerve, that we interpret as sound.”
“Oh wow,” replied Simon. “So when we hear sound, it’s actually microscopic hairs bending in fluid inside of our ears. But what does this have to do with balance?”
How our ears help us know how we are moving
“We’re getting there!” replied Dr. Tanaka, reassuringly. “To understand how balance and the righting reflex work, you need to know about the semicircular canals, utriculus and sacculus. Together, these structures make up the vestibular system, which helps our brain perceive how and whether we are moving.”
“So it looks like this vestibular system is actually a part of the cochlea? Or that they’re connected somehow?” remarked Simon, with a puzzled expression.
“They are indeed!” replied Dr. Tanaka. “Remember the hair cells in the cochlea that detect sound by bending when the fluid around them moves? Well, the utriculus, sacculus, and semicircular canals all have hair cells too, similar to those in the cochlea. And the same fluid that fills the cochlea also fills the vestibular system.
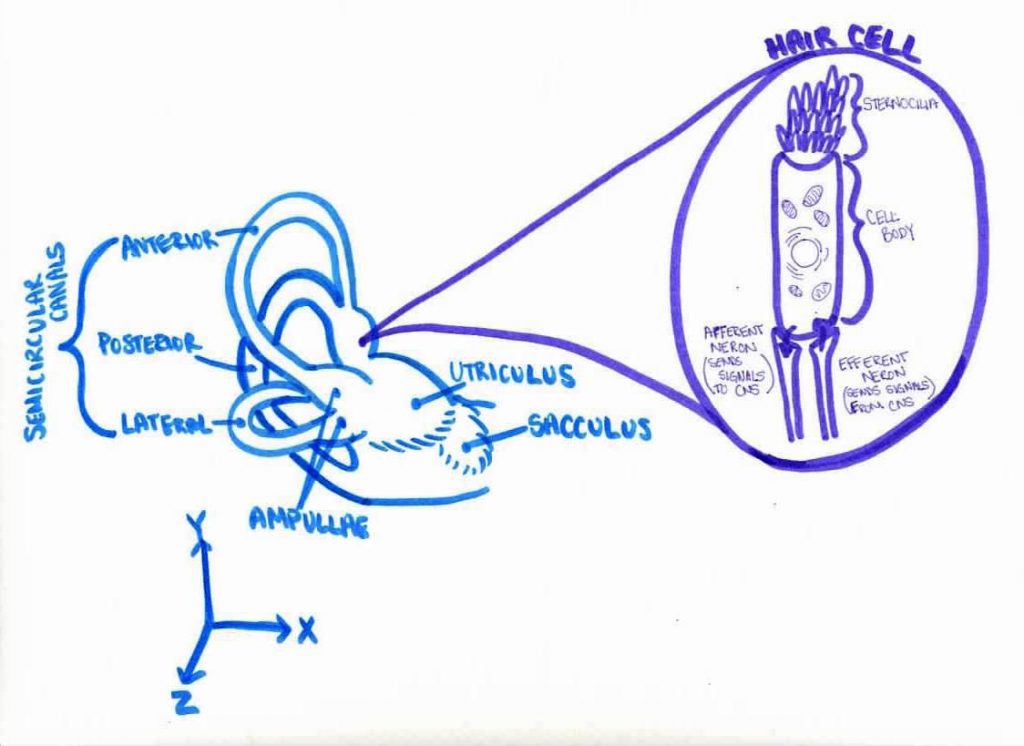
“The hair cells in the vestibular system work slightly differently but the basic principle is the same,” continued Dr. Tanaka. “At the base of each semicircular canal the canal widens into to form an ‘ampulla’ and inside this ampula are hair cells embedded in a kind of jelly called a cupula. If you go from standing still to spinning in a particular direction, that is if you accelerate while rotating, the fluid inside the semicircular canal starts to spin too and this causes the jelly cupula to deform and the hairs inside it to bend.”
“Oh and just like you detect sound vibrations from the bending of the hair cells in the cochlea, you detect when you’re spinning from bending of the hair cells in the ampulla of the semicircular canal?” asked Simon.
“Exactly! And specifically, the semicircular canals are detecting ‘angular acceleration’, that is if you’re speeding up or slowing down while spinning.”
“Ah I see,” replied Simon, nodding slowly. “But wait, why are there three semicircular canals in your drawing? Isn’t that redundant?”
“Great question! Each semicircular canal can only detect acceleration in the direction the fluid is flowing through the loop or along a single axis. But we live in a three dimensional world with three axes so we need three loops to detect rotational acceleration in three dimensions. You can think of them kind of like having an X, Y, and Z axes in your ears. That’s why there are three loops oriented at 90 degrees relative to one another.”
“I see. So it’s like we have this gyroscope in our head with three loops for determining rotational acceleration along each axis,” remarked Simon.
“Well, we actually have two gyroscopes, if you think about it! One on the left and one on the right,” replied Dr. Tanaka. “Having semicircular canals on both sides of our head helps us determine the direction in which we are accelerating as we spin. The neurons connected to the hair cells in the semicircular canals don’t send a signal just when the hairs are bent but when they bend in a particular direction. Since the fluid spins in opposite directions on each side, the brain can tell the direction in which we’re accelerating based on whether the neural signal is coming from the left or the right side.”
“Oh wow. I never realized we have such a complicated navigational system in our head!” Simon replied, reanimating a toy mouse on a string to keep Grace entertained. “So, if Grace starts spinning through the air as she falls then the semicircular canals can help her figure out the direction in which she’s spinning. But how does she know she’s falling down, since she’s falling in a straight line, not rotating? And how does she know which way is up so she can position her feet under her?”
“Ah, yes. Remember how I mentioned there are also hair cells in the utriculus and sacculus of the vestibular system?” Dr. Tanaka asked, pointing to her drawing. “The hair cells in the utriculus and sacculus can sense if you’re speeding up in a straight line, that is linear acceleration.”
“How do they do that?”
“The utriculus and sacculus have tiny, dense rocks called otoliths made out of calcium carbonate, the same material that makes up limestone and sea shells. These are embedded on top of a membrane that encloses the hairs of the hair cells, like the jelly cupulas in the semicircular canals. Because the otoliths are heavier than the membrane they’re embedded in, they move more slowly than the membrane if you start to accelerate in a particular direction…”
“And this causes the hairs embedded in the membrane to bend!” Interrupted Simon.
“Exactly! You’re getting the hang of it now,” Dr. Tanaka said smiling. “And recall that gravity is simply a linear acceleration toward the ground. So even when you’re standing still, the otoliths will fall just a bit toward the ground due to gravity, deforming the membrane they’re embedded in and telling you which direction is down. The utriculus and sacculus are also oriented at 90 degrees to each other so they can sense linear acceleration in any direction.”
“Ah, I see,” replied Simon. “The semicircular canals help Grace know if her head starts spinning in a particular direction and the utriculus and sacculus help her know if she starts falling to the ground and which way is up.”
“Yes! We humans have the exact same vestibular system. But because cats have such a flexible spine, they can use this information about how they’re spinning and where the ground is to rotate the front half of their body differently from the back half and get both their legs under them midair.”
What can go wrong
Just as Dr. Tanaka had finished speaking, an older cat wandered unsteadily into the exam room and meowed. “Hey there Whiskey,” Dr. Tanaka spoke kindly, “have you come to say ‘hello’ to Grace? Whiskey here is our clinic pet. But he’s a bit unsteady on his feet,” Dr. Tanaka said as he patted Whiskey’s head, just behind his ears.
“Oh no. Is there something wrong with his inner ear?” asked Simon.
“Yes, unfortunately,” replied Dr. Tanaka. “What we think happened is that some of his otoliths have become detached from the membrane in the sacculus and utriculus. These free floating otoliths can interrupt the normal bending of the hair cells and cause vertigo, or a loss of balance. Other than walking funny, he seems to be able to do everything else just fine. But he’s learned not to leave the floor.”
“Yeah, I guess he wouldn’t be able to figure out where the ground was for his righting reflex!” remarked Simon.
“Yes, exactly. The same thing can happen in humans too and cause vertigo,” noted Dr. Tanaka.
“Oh, is that why I sometimes get dizzy on the rides at the amusement park or on a boat? Is that dangerous?”
“Ah, no that’s totally fine,” replied Dr. Tanaka reassuringly. “When you feel seasick or dizzy from being on a ride or a boat your otoliths are where they are supposed to be. But your brain gets confused by your frame of reference. Your vestibular system senses that you’re bouncing up and down. But according to your eyes, everything on the ride and the boat are standing still. Your brain is unaccustomed to processing this conflicting information so the result is that you feel queasy.”
“Oh OK, good! I don’t want to stop riding amusement park rides.”
“Speaking of amusement, I think that Grace is getting a bit restless. How about we get started with her check-up?”
“Yes I think you’re right- I’m surprised she’s stayed entertained this long. And thank you for explaining balance to me. I had no idea that hearing and balance were so closely related. All thanks to the tiny hair cells!”
Written & illustrated by Kelly Diamond, PhD
Edited by Aaron Olsen, PhD
While the scientific content in this post is not fictional, the names, characters, events and incidents are; any resemblance to actual persons, living or dead, or actual events is purely coincidental. Except where otherwise indicated, © 2021 3D Anatomy Studios. All rights reserved.