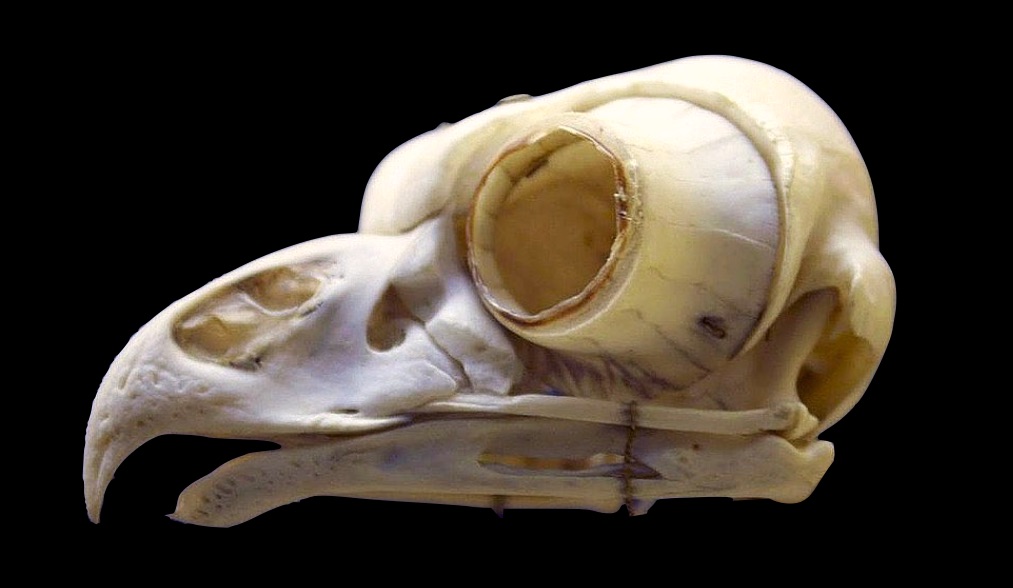
“Aunt Iesha, are these rings of bones? In their eyes?” Rana and her aunt were walking through the Bone Hall on their way to the Smithsonian café.
“What’s that Rana?” her aunt asked, turning around and walking to her niece’s side to see what Rana was pointing at. On the other side of the glass, the skeletons of a grebe, heron, and loon stared back at them. “Is it optometry school now?” her aunt asked, smiling at her niece. Her niece had always been a curious kid, asking her curator aunt questions, generously assuming her aunt’s area of expertise must surely encompass all of science. But lately Iesha had made a game of guessing Rana’s ever changing future career ideas from the questions she asked.
Rana smiled back, “Yeah, you got me. We learned about the eye in A&P a few weeks ago and ever since it’s been on my mind. Like, it’s just this fascinating mix of physics and biology. I don’t remember there being any bones in the eye though.”
“Well you are right about that, humans don’t have bones in their eyes. But most vertebrates do.”
“Thank goodness. That’s two fewer bones I have to learn in A&P,” Rana said, laughing with relief. “But if most vertebrates have these bones, and humans are vertebrates….”
Her aunt started to smile and nod, “Then why don’t we have bones in our eyes?”
Exactly,” Rana confirmed, beaming at the recognition of how much they could communicate without words.
“That is a great question, Rana,” a full smile breaking on her aunt’s face. “But I need tea first. Let’s go downstairs and I’ll tell you more than you want to know.”
“I wouldn’t expect anything less from you!” Rana replied laughing.
Why is the eye not just a retina?
Tea and pastries in hand, Rana and her aunt sat down at a table in the corner of the atrium, light and voices echoing overhead. Before even taking a sip, her aunt pulled out a notebook and pencil.
“You’d forget your keys and wallet before you’d forget your notebook and pencil!” Rana joked, wasting no time in taking a first bite of her pastry. After only a brief concurring smile, her aunt pursed her lips as she flipped to a new page in the notebook to start drawing.
“So you understand how a human eye works, right?”
“We just had that test last week!” Rana replied, in a language she knows her aunt will understand.
“So, that’s a ‘no’ then,” her aunt said with a knowing grin.
“Exactly. It’s gone already,” Rana said, waving her hand back over her shoulder.
“Well,” her aunt started, “the eye is all about taking light that comes into the eye from the outside through the pupil and focusing it onto a layer of light sensitive cells inside the eye.”
“The retina.”
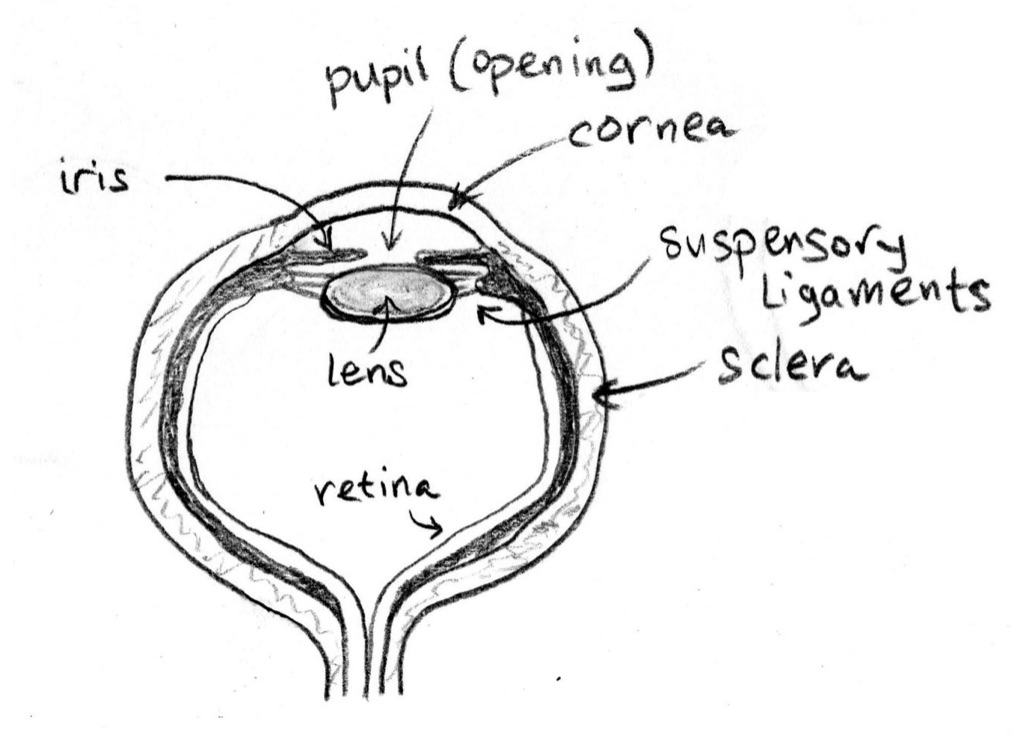
“That’s right. The retina is this sheet of neurons at the back of the eye that fire only when a certain intensity or even color of light hits them. The outside world is literally projected through your eye onto this layer of cells and your brain puts together an image based on which neurons are firing and which ones aren’t.”
“The neurons are called photoreceptors, right?”
“That’s right. Because they are ‘receptive’ or responsive to photons, the particles that make up light.”
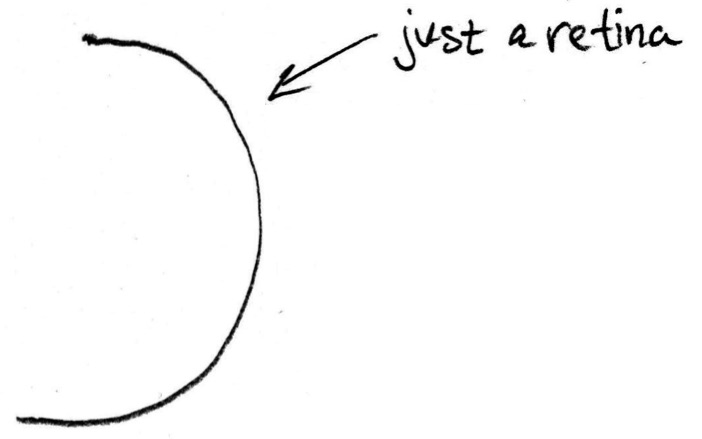
“OK, so if the retina is made up of photoreceptors that can turn on and off based on the light that is projected onto them, why don’t we —and maybe this is a ridiculous question— just have the retina? The light from outside would simply come in, hit the retina, and that’s what we’d see.”
“That’s not a ridiculous question at all! Some animals actually have exactly that, simply a layer of cells that are sensitive to light. The problem is —and here you have to think about the fact that our external environment is flooded with light rays bouncing every which way— those light sensing cells get bombarded with light from every angle and every direction. The image is totally blurred because every light wave, or photon, can hit those cells.
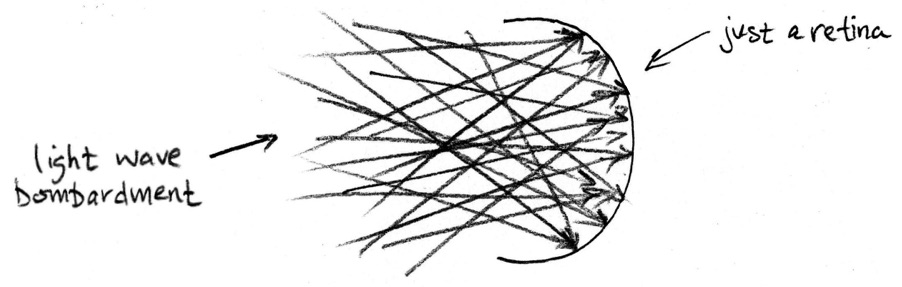
“So a simple layer of light sensing cells is useful if you just need to know how much light there is and where it’s coming from. There are tiny aquatic animals called flatworms that have an ‘eye’ just like that for swimming toward or away from light.”
“I see,” said Rana, nodding slowly, her eyes focused in concentration as she removed the bag from her tea before it got too bitter. “To see a clear image, there needs to be a way to limit or control how light enters the eye and hits the retina?”
“Yeah, that’s a good way to put it. Another way I like to think about it is, in order to get a clear image on the retina, all the light coming from a single point outside the eye, such as the tip of this pencil, has to converge on a single point on the retina. If you repeat that for a series of points making up some object, like points all over this pencil, then you’ll be able to see the whole pencil clearly on the retina.”
How does a pinhole camera work?
“Oh, you know what this is making me think of? That experiment we did in school when I was a kid. Something like a shoebox camera?” asked Rana, eyes squinted and index finger pressed to her chin.
“Ah, yes! You made a pinhole camera. Those are a lot of fun to make. You create a little aluminum window at one end of the box to poke a tiny hole through using a sewing needle, the aperture or opening of your camera.”
“And then a piece of translucent plastic on the other side as a screen to see the image that’s projected through the pinhole,” finished Rana.
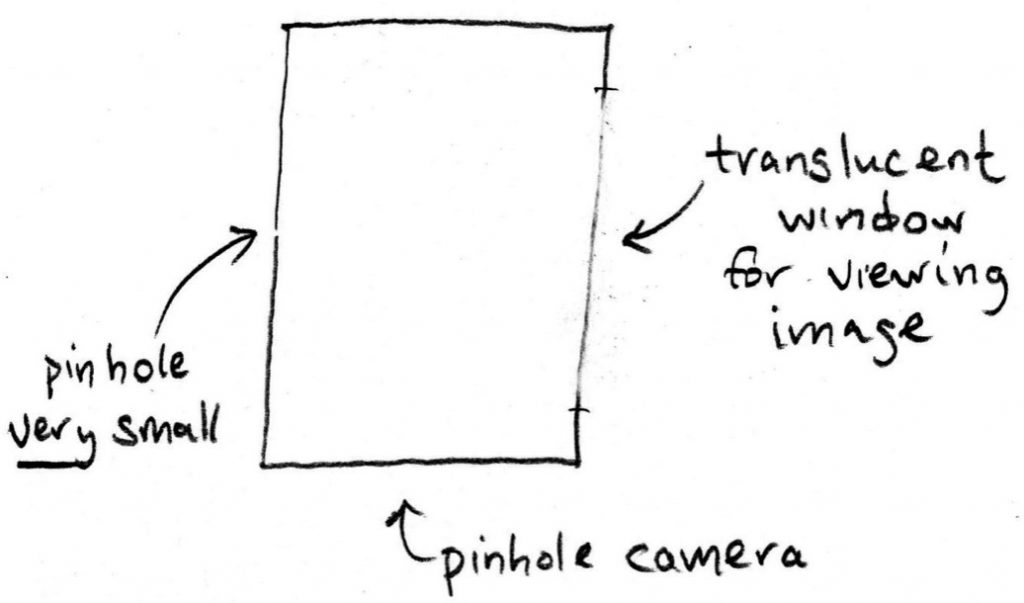
“Exactly. So that’s actually one way you can get a clear image. The pinhole is so small, just a fraction of a millimeter, that for any point outside the camera, only light rays traveling directly toward the pinhole can enter. So you get that one-to-one correspondence of points between the outside and the inside of the camera that gives you a clear image. And the distance between the pin hole and the screen doesn’t matter. The image gets larger or smaller, but it’s always in focus.”
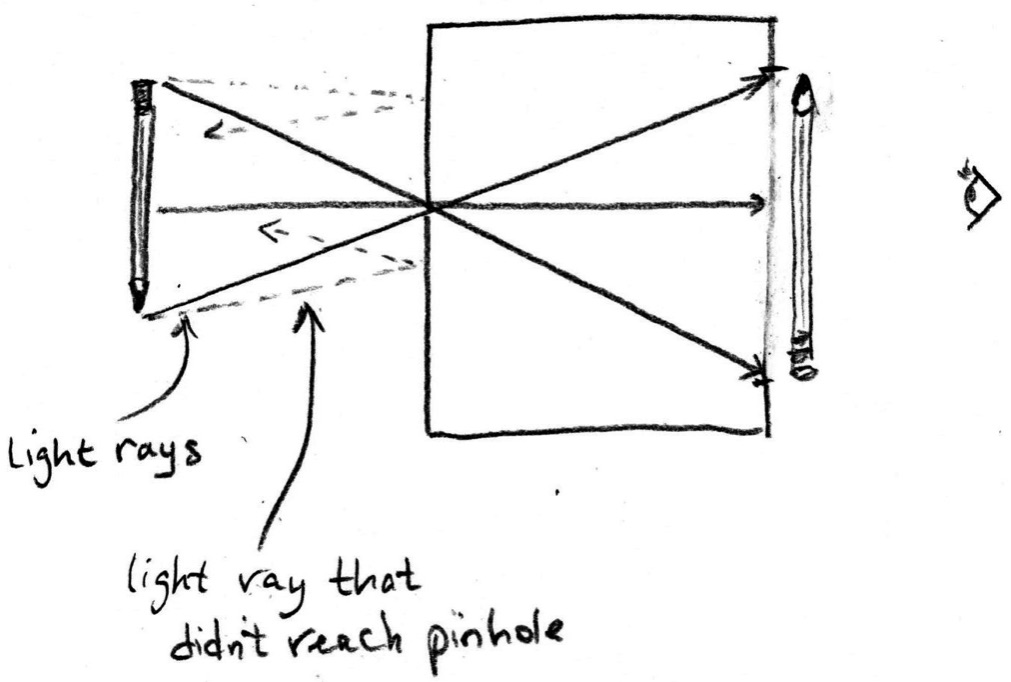
“OK, I see. So the human eye is like a pinhole camera, the pupil is the pinhole, the transparent screen is the retina….”
“Well, think about that for a minute,” her aunt interrupted, holding up her hand and taking a sip of tea. “How big is your pupil relative to the pinhole you created for your shoebox camera?”
“Hmm,” Rana said, squinting slightly as she looked into her aunt’s eyes for reference. “Oh, I see, our pupils are much larger.”
“That’s right, our pupils are over 10 times larger than the size of the hole in a pinhole camera. If you made your shoebox camera’s pinhole that large, your image would be totally blurry because for any point outside the camera you would get a spread of points across the screen. Do you remember how bright the image in your pinhole camera was?”
“It was actually really hard to see! It was so dim.”
“Exactly. Hardly any light can get in through a hole that small. There are actually animals that have eyes like a pinhole camera, the nautilus, for example. But the image on their retinas is very dim. If our eyes worked like that we would have a really hard time seeing anything, except in extremely bright light.”
“So the pupil,” Rana replies, with one hand outstretched and her eyebrows furrowed, “isn’t like a pinhole in a shoebox camera. It’s much too large for that. But wait, why do we have a pupil then?”
“Ah yes, now you see how these analogies, like a shoebox camera, can be really useful for understanding a basic phenomenon, like how light rays travel. But they can trip you up if you try to apply them directly to biology, where you have multiple complicated phenomena all interacting with each other. Because the pupil is too large to function as a pinhole, it simply functions like window blinds for the eye, regulating how much light can get into the eye. Those photoreceptors in the retina are very sensitive and too much light will damage them. So when it’s too bright the pupil can close to protect the retina.”
If the human eye doesn’t work like a pinhole camera, how does it work?
“OK, so if our eyes don’t work like a pinhole camera to get a clear image, how do we get that one-to-one correspondence on our retina?”
“Ah, yes. That’s where the cornea and lens come in. The cornea and lens take all of the light coming from a single point outside the eye and ‘bend’, or refract, it toward a single point on the retina. Do you remember how refraction works?”
“I remember how when you put a pencil in a glass of water, it looks like the pencil is broken. That’s refraction, right?”
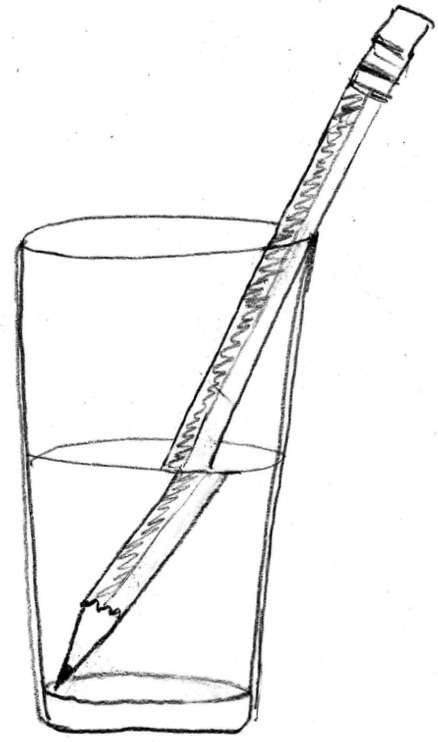
“Yes, exactly. Refraction is all about light speeding up or slowing down at the interface between two materials. Light doesn’t travel at the same speed through all materials. For example, it travels more slowly through water than through air. So if light is traveling through air and then hits water at any angle other than ‘head on’, it will change direction or be ‘refracted.’ It keeps traveling in a straight line —that is, it doesn’t ‘curve,’ so the word ‘bend’ can be a bit confusing. But it does change direction.
“The property that tells you how fast light travels through a material is the ‘refractive index’ and water has a refractive index of 1.33, while air has an index of one. That’s why the pencil looks like it’s broken where it enters the water. The light waves bouncing off the pencil below the water have been refracted at a different angle relative to the waves bouncing off the pencil in air, above the water. So refraction depends on the difference in refractive index between the two materials at an interface and the angle at which the light hits that interface.”
“Oh, I see. So the light actually gets refracted before it hits the lens, when it hits the fluid in the eye?”
“You’re thinking of the aqueous humor, the fluid in the anterior chamber of the eye, just behind the cornea?” her aunt asks, her eyebrows raised.
“Yes?” Rana replied, with a measure of doubt after considering the expression on her aunt’s face.
“Well, remember that the human body is mostly water and that’s true of all the structures in the eye, including the first structure light passes through, the cornea. In fact, the cornea, the fluid in the anterior chamber of the eye (the aqueous humor), and the lens all have an index nearly identical to that of water.”
“So most of the refraction happens when the light first enters the eye through the cornea. When it goes from air into these water filled tissues,” interjected Rana, quick to correct her previous reply.
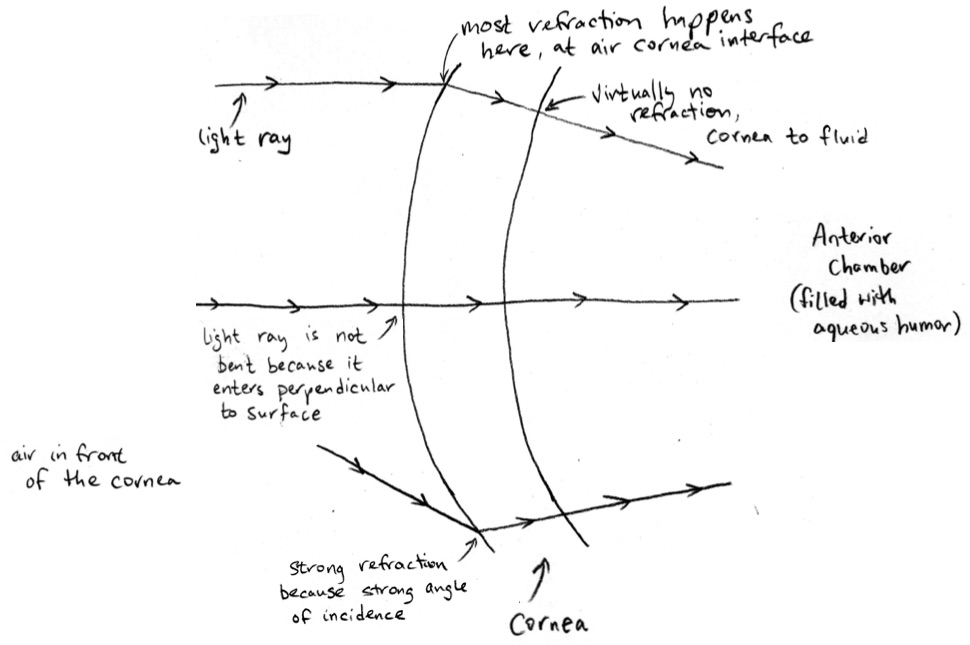
“Now you’ve got it,” her aunt said, smiling. “The refractive index of the lens isn’t exactly the same as the fluid in front and in back of it, so the lens also has some refractive power. In humans, 20% of the refraction happens in the lens and 80% happens at the air-cornea interface. But of course, once you go underwater light is no longer traveling from air to water-filled tissue, it’s water to water-filled tissue. So vertebrates that have to see well underwater have lenses with greater refractive power.”
How do vertebrate animals focus?
“Oh wow, yes, I hadn’t thought about that,” replied Rana. “So with a pinhole camera, the image is always in focus. But why when I focus on my cup of tea, for example, is most everything else around me blurry?”
“Ah, yes. That’s a trade-off. By using our cornea and lens to refract light we’re able to get much more light into our eye than for a pinhole camera. But the downside is that not everything is in focus at the same time. For example, all the light coming from your cup of tea is refracted to a particular point inside the eye called the focal point. Since your tea is in focus, that focal point must be at the retina. But if you maintain focus on your tea, light rays coming from your pastry, which is closer to you, are meeting at a focal point behind your retina. And light rays from my pencil, which is further away, are meeting at a focal point in front of your retina.”
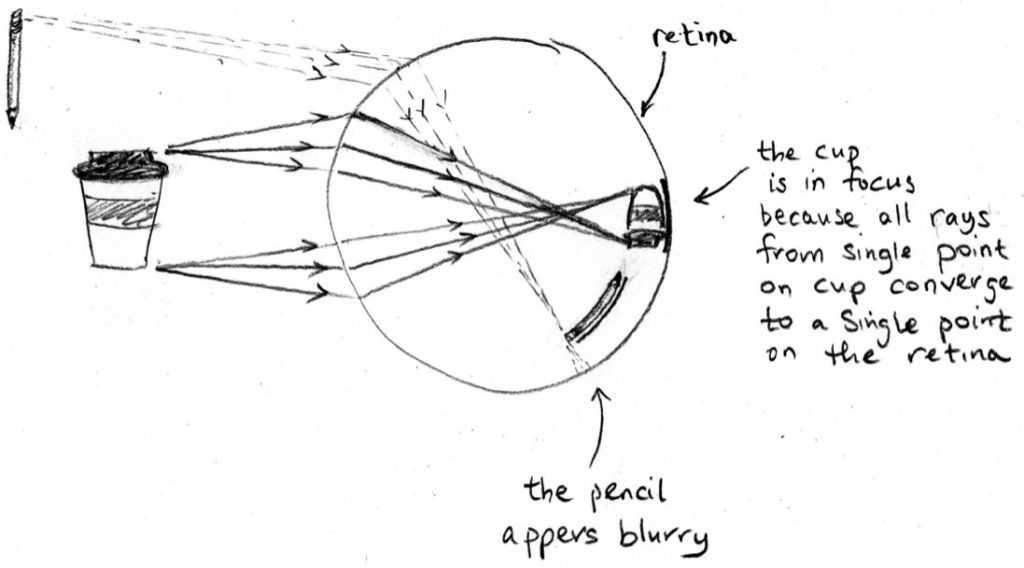
“Hmm, but I’m able to change what I’m focusing on, if I want to.”
“Thankfully, yes!” chuckled her aunt. “Or else you’d have to walk toward and away something every time you wanted to focus on it.”
“That would look pretty silly.”
“Yes, it would! Changing what your eye is focused on is called ‘accommodation’ and what you’re doing is changing how the light is refracted in the eye to move that focal point into alignment with the retina. Remember that refraction at the interface of two materials depends on the difference in refractive index between those two materials and the angle at which the light hits the interface. You can’t change the refractive index of the cornea or lens but you can change the angle the light hits them at by bending them and changing their curvature or moving them relative to one another.”
“Ah, right,” replied Rana, nodding. “I’m remembering now how there’s that muscle that can cause the lens to either bulge out or flatten.”
“Yes, that’s exactly how humans and other mammals focus,” confirmed her aunt. “The lens is suspended just behind the pupil by a ring of suspensory ligaments, like a trampoline suspended by springs. And where these suspensory ligaments are anchored in the eye is a ring of muscle called the ciliary muscle. You might think that when this muscle contracts it causes the lens to flatten, but it’s actually the opposite.”
“Oh, yeah. I think I actually messed up that question on the test,” admitted Rana. “But I remember now, of course,” she said laughing. “When the ciliary muscle contracts, it shrinks that ring, releasing tension in the suspensory ligaments and causing the lens to bulge. Like shrinking the ring around the trampoline, the trampoline would pull in on itself. And bulging of the lens increases its curvature, allowing you to focus on closer objects.”
“Exactly. But changing the shape of the lens is not the only way vertebrate animals focus. For example, amphibians, many types of fish, and possibly even snakes focus by actually moving their lens forward and backward within the eye. They have a different muscle in their eye to do this, the retractor and protractor lentis muscle. This effectively changes the distance between the lens and the retina. And birds and lizards can also deform their cornea to focus by contracting their ciliary muscle, since this muscle forms a ring at the base of the cornea. Many of these vertebrates use multiple types of accommodation, whereas mammals, or mammals who have the ability to focus, just change the shape of their lens.”
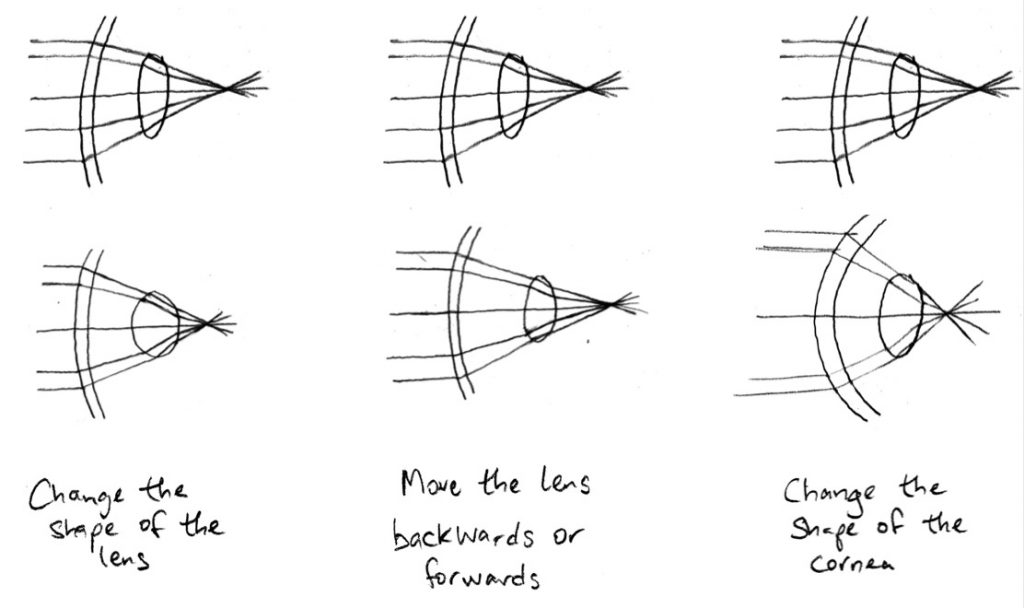
“How boring we are!” laughed Rana. “But in a way, we as humans also change the shape of our corneas to focus.”
“We do?” asked her aunt, surprised.
“Well, when I got contact lenses or when my dad got lasik surgery, we’re essentially changing the shape of our cornea, like a bird or a lizard.”
With a smile growing on her face, her aunt replied, “Well, that’s true isn’t it? Although we can’t change our focus dynamically that way, it does use the same principle.”
Why don’t humans have bones in their eyes?
As Rana stared off to one side, her eyebrows furrowed and her lips pursed to one side, she said, “So we’ve talked about how our eyes and the eyes of other vertebrates have all these ways to make sure a clear image is projected onto the retina. But I’m imagining an animal focusing, contracting this ring of muscle inside their eye to change the shape or the lens or the cornea, or contracting those muscles that move the lens back and forth. If the eyeball is just this, well, squishy ball, wouldn’t all those muscles contracting cause the whole eye to deform, including the retina, and distort the image?”
Smiling, her aunt replied, “And that brings us to the rings of bone that you pointed out in the bird skeletons earlier! Most vertebrates have what’s called an ‘ocular skeleton,’ composed of cartilage and bone, embedded in the outermost layer of their eye.”
“That layer’s called the sclera, right?”
“Exactly. And since the bone portion of the ocular skeleton is embedded in the sclera, the bones are called ‘scleral ossicles.’ Most fishes, birds, lizards, and turtles have some kind of ocular skeleton. We mammals, except for monotremes like the platypus and echidna, are the exception in not having them.”
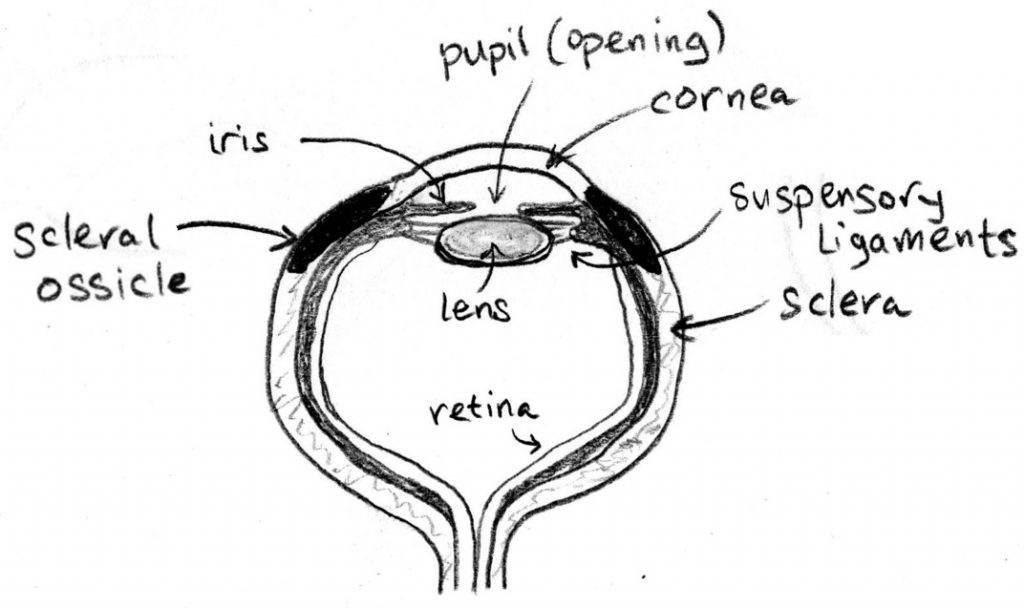
“So having this ocular skeleton of cartilage and bone embedded in the sclera is what stiffens the eyeball in most vertebrates and prevents the retina from being distorted as the internal muscles are deforming the lens and cornea during focusing?”
“That’s the best explanation we have so far. If you look at vertebrates that fly really quickly —animals we know really need to change their focus quickly— or animals that swim really fast under water, where there can be big pressure changes on the eye because of that speed, they have especially strong ocular skeletons. And fish that have evolved to the point where they no longer need to see, like fish that live in pitch black caves, have lost their ocular skeleton completely.”
“But,” started Rana, staring off with a puzzled expression, “I think of mammals as generally having good vision. And there are mammals that run, fly, and swim quickly. It seems strange that, except for platypus and echidna, no mammals have an ocular skeleton?”
“We actually don’t completely understand why mammals don’t have an ocular skeleton.”
“Wait, are you saying these ocular skeletons are like cutting-edge research?” Rana said, incredulous.
“Very much so,” her aunt said, laughing. “There’s still a lot we don’t understand about how other vertebrate eyes work. For starters, it looks like the ocular skeleton was lost long ago in mammal evolution. And once something has been lost, it can be hard to re-evolve. So part of the answer for why humans don’t have an ocular skeleton is simply that all but just a handful of mammals don’t have one, and humans are mammals.”
“Ah, like it’s a trait that we simply inherited from our ancestors,” replied Rana.
“Exactly. But there are a few hypotheses as to how mammals may be compensating for the lack of a skeleton. For example, one hypothesis is that mammals who need a stiffer eye simply have a tougher or thicker sclera, rather than having cartilage or bone. The sclera and cornea are made of collagen, the same protein in tendons and ligaments, which can be very fibrous.
“Another hypothesis is that because most mammals have a spherical eyeball, it can better resist the forces from the internal muscles during accommodation. Vertebrates that have ocular skeletons tend to have non-spherical eyeballs, which are better at resisting forces in some directions than others.
“And remember how there are three ways that vertebrates focus —deforming the cornea, moving the lens, and deforming the lens— but mammals only deform their lens? Another hypothesis is that if all you’re doing to focus is changing the shape of your lens, you’re not putting so much stress on the eye that you need an ocular skeleton.”
“Huh,” Rana wondered aloud, “I never would have thought about the human eyeball being so tough because it’s compensating for not having an ocular skeleton. Like, I wouldn’t have thought a structure was ‘missing’ if I didn’t know about how eyes work in other vertebrates.”
“And that’s why if you continue down a career path in human health, Rana, you should always think about it in the context of vertebrate or animal evolution and diversity. If not, it’s like not knowing anything about the history of your culture, or the history of other cultures; you think ‘things are this way simply because that’s the way they are.’ Seeing human health in an evolutionary context helps you understand that where we are today is a product of everything that came before us. And understanding human health in the context of vertebrate diversity helps you understand the fundamental problems that our bodies must solve, like preventing the actions of the muscles inside the eye from deforming the eye so that our vision isn’t distorted. And that there are many, equally valid solutions we are just beginning to understand.”
“You’re not trying to get me to become a curator too, are you?” Rana asked, laughing.
“Would that be so bad?” her aunt replied, smiling.
Written & illustrated by Aaron Olsen, PhD
Edited by Kelly Diamond, PhD
We would like to acknowledge the article Skeletons of the Eye: An Evolutionary and Developmental Perspective by Tamara A Franz-Odendaal, published in 2020 in The Anatomical Record; it was an invaluable source of information in preparing this piece. While the scientific content in this post is not fictional, the names, characters, events and incidents are; any resemblance to actual persons, living or dead, or actual events is purely coincidental. Except where otherwise indicated, © 2021 3D Anatomy Studios. All rights reserved.
Have a question or comment? Please leave it below!